
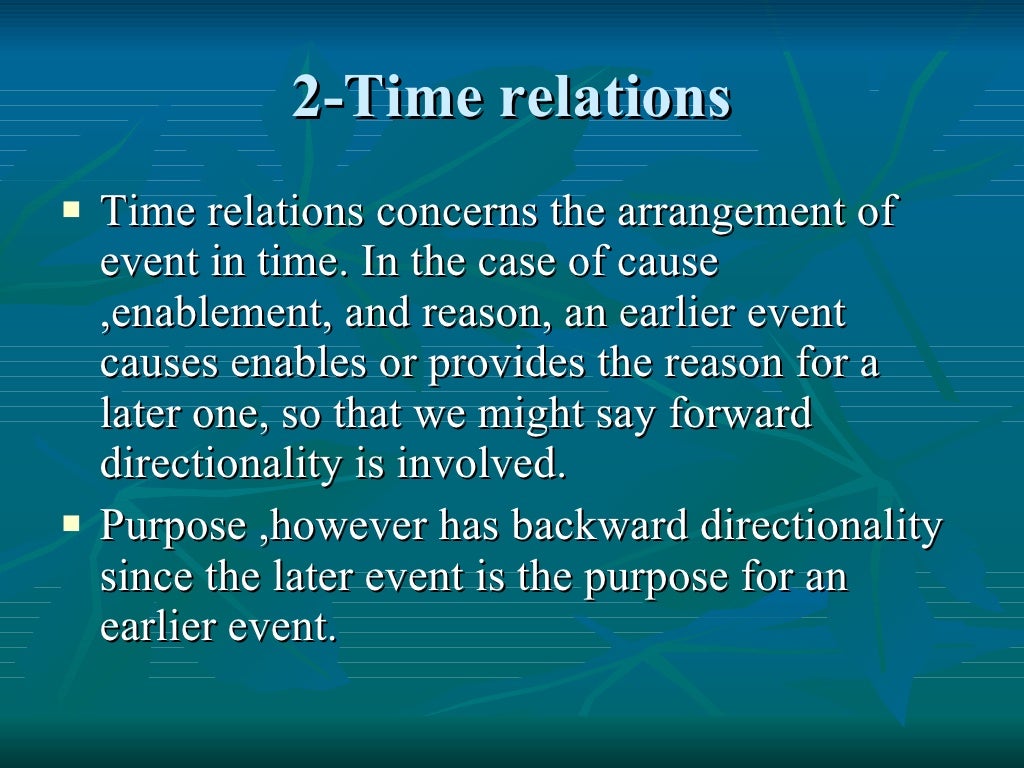
This diagram shows three heartbeats recorded on an electrocardiogram (ECG). Heart rate variability is a measure of the beat-to-beat changes in heart rate. This naturally occurring beat-to-beat variation in heart rate is called heart rate variability (HRV). Rather than being monotonously regular, the rhythm of a healthy heart-even under resting conditions – is actually surprisingly irregular, with the time interval between consecutive heartbeats constantly changing. Scientists and physicians now know, however, that this is far from the case. The heart at rest was once thought to operate much like a metronome, faithfully beating out a regular, steady rhythm. This means that learning to generate increased heart rhythm coherence, by sustaining positive emotions, not only benefits the entire body, but also profoundly affects how we perceive, think, feel, and perform. In contrast, the more ordered and stable pattern of the heart’s input to the brain during positive emotional states has the opposite effect – it facilitates cognitive function and reinforces positive feelings and emotional stability. (This helps explain why we may often act impulsively and unwisely when we’re under stress.) The heart’s input to the brain during stressful or negative emotions also has a profound effect on the brain’s emotional processes-actually serving to reinforce the emotional experience of stress.
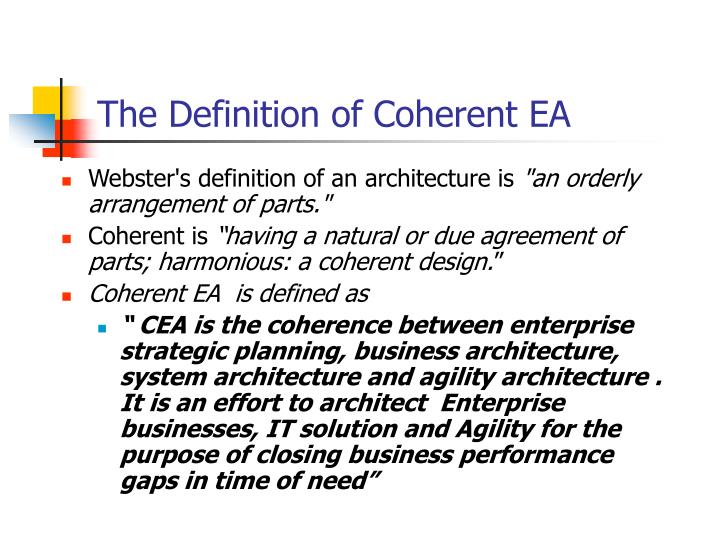
This limits our ability to think clearly, remember, learn, reason, and make effective decisions. During stress and negative emotions, when the heart rhythm pattern is erratic and disordered, the corresponding pattern of neural signals traveling from the heart to the brain inhibits higher cognitive functions. HeartMath Institute research has demonstrated that different patterns of heart activity (which accompany different emotional states) have distinct effects on cognitive and emotional function. Scientists at the HeartMath Institute have extended this body of scientific research by looking at how larger-scale patterns of heart activity affect the brain’s functioning. Earlier research mainly examined the effects of heart activity occurring on a very short time scale – over several consecutive heartbeats at maximum. The effect of heart activity on brain function has been researched extensively over about the past 40 years. In other words, not only does the heart respond to the brain, but the brain continuously responds to the heart. However, it is not as commonly known that the heart actually sends more signals to the brain than the brain sends to the heart! Moreover, these heart signals have a significant effect on brain function – influencing emotional processing as well as higher cognitive faculties such as attention, perception, memory, and problem-solving. Most of us have been taught in school that the heart is constantly responding to “orders” sent by the brain in the form of neural signals. The Resilient Heart™ – Trauma-Sensitive.Young's experiment with single photons.Introduction to Young's experiment, in which we explain Young's experiment and calculate the intensity as a function of angle.On the other hand, if we specify the wavelength precisely to ensure strong interference, then we can't talk of one photon being reflected much before the other. Via the uncertainty principle, this means that we don't know the wavelength well, so interference will not be strong. The short answer is this: When we say 'striking' or 'reaching' an interface, and that one has reflected before the other, we imply that we can define the times of arrival very precisely, which means a very a very short coherence length and so little interference.Īlternatively: Talking about particles implies localisation in space. How can it later be cancelled out by a photon that hasn't even reached the second interface yet? Suppose that one photon is reflected at the first interface. Here's one way of putting it: Consider photons striking a thin film, as in our animation, and producing destructive interference. Remember the question in the multimedia tutorials Interference. However, those that have travelled further need not have a correlated phase, because they were emitted by a different event that occurred earlier. In practice, there are usually many photons simultaneously. Here there is no overlap and so no interference. However, this is longer than the coherence length of the light in our cartoon, which is again 2 λ. In the second cartoon, the difference in optical pathlength is 2 nt = 5λ/2, which would produce destructive interference for light from a highly coherent source. Optical path length difference shorter than the coherence length
